Understanding Soil Compression: Implications and Techniques
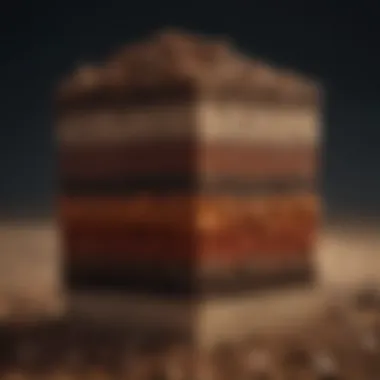
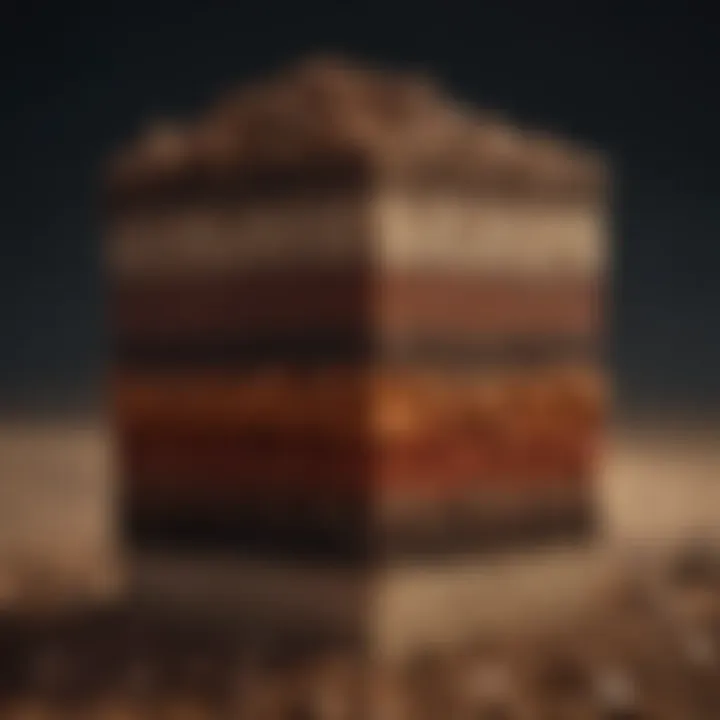
Intro
Soil compression refers to the process in which soil particles are packed closer together, often leading to a decrease in soil volume and porosity. This phenomenon affects how water, air, and nutrients move through the soil, subsequently influencing plant growth and agricultural productivity. Understanding this concept is not just useful; it is indispensable for anyone operating within the realms of agriculture, horticulture, or agronomy.
In agriculture, soil compression can translate into a double-edged sword. On one hand, it may enhance stability and load-bearing capacity for structures like greenhouses or fences. On the flip side, too much compression can spell disaster for crops due to poor drainage, root development issues, and limited nutrient availability. Whether you’re a seasoned farmer or someone with a budding interest in plants, grasping the nuances of soil compression will equip you with the insight needed to implement effective management practices.
Understanding the balances and trade-offs involved in soil compression informs the strategies used to maintain healthier soil and crops. This article embarks on a journey through the various facets of soil compression, encompassing its causes, impacts, and the range of methods available for assessment and mitigation.
What is Soil Compression?
Soil compression is a fundamental concept that stands at the crossroads of agriculture, engineering, and environmental science. It refers to the process by which soil particles are packed more closely together, resulting in a decrease in soil volume. This phenomenon has far-reaching implications, influencing everything from crop growth to the integrity of buildings and roads. Understanding soil compression isn't just an academic pursuit; it's an essential component for anyone involved in farming, land management, or construction.
As we delve deeper into this topic, we'll uncover the critical aspects of soil compression. This exploration can greatly inform the practices of farmers, agronomists, and land developers, ultimately leading to more sustainable and effective use of soil resources.
Definition and Key Concepts
Soil compression can be defined as the reduction in volume of the soil mass caused by applied loads. When external forces, such as weight from buildings or heavy machinery, exert pressure on the ground, the spaces between soil particles, known as voids, decrease in size. This process can lead to several important phenomena:
- Increase in Density: As soil is compressed, it becomes denser, which can affect its properties.
- Decrease in Permeability: Compacted soil can lead to reduced water flow, impacting drainage and the roots' ability to absorb moisture.
- Alteration in Mechanical Properties: The strength and stability of soil can change significantly due to compression.
It’s important to note that the degree of soil compression can vary greatly depending on soil types—clay, sandy, or silty—each responding differently to compressive forces.
Historical Overview
The understanding of soil compression has evolved over centuries, intertwined with advancements in agricultural practices and civil engineering. Historically, the knowledge of how soil behaves under load dates back to ancient civilizations. The Egyptians, for example, had a keen awareness of soil's weight-bearing abilities when constructing monumental edifices like the pyramids. They understood that not all soils were created equal and that certain compaction techniques were necessary to support heavy structures.
Fast forward to the Industrial Revolution, which brought about significant changes in land use and farming techniques. With the rise of machinery, soil compression became a pressing concern. Farmers began to notice that heavy tractors and harvesters compacted their fields, leading to less crop yield—a lesson that was learned the hard way over time. This pivotal moment ignited further research into soil science, encouraging the development of methods and materials designed to mitigate compression effects.
In the modern era, the advent of technology has enhanced our understanding of soil behavior. Tools for soil testing and modeling have made it easier to predict soil responses to various stresses, ultimately shaping better practices in agriculture and construction. As the dialogue surrounding sustainable practices gains momentum, understanding soil compression gains even more significance, prompting ongoing studies and innovations aimed at preserving soil health while optimizing land use.
"Healthy soil is the foundation for healthy plants and can significantly improve the yield and sustainability of agricultural practices."
Mechanisms of Soil Compression
Soil compression is not just a thing of curiosity; it’s a fundamental aspect that affects agriculture, urban planning, and environmental science. Understanding the mechanisms behind soil compression allows for better decision-making and planning in various fields, from farming practices to construction. Different types of soil respond diversely to compressive forces; knowing these reactions aids in predicting and managing soil behavior effectively, especially when heavy structures are involved or when dealing with natural landscapes.
Types of Soil and Their Response
Clay Soils
Clay soils are known for their fine, dense particles. They have a unique characteristic that makes them more sensitive to compression. When it comes to compressive forces, their plasticity and cohesion allow them to change shape without cracking, making them pretty adaptable in certain conditions. In agricultural settings, clay soils can hold water and nutrients well, which lends itself to healthier crops. However, this same quality can turn into a double-edged sword; excess compression can lead to poor drainage and root issues.
Benefits:
- Excellent moisture retention, benefiting crops during dry spells.
- Provides vital nutrients, essential for plant growth.
Disadvantages:
- Can become compacted easily, hindering root growth and access to oxygen.
- Poor drainage can lead to waterlogging, especially during heavy rains.
Sandy Soils
Sandy soils, on the flip side, are known for their larger particles and excellent drainage capabilities. This type of soil is characterized by its loose composition which results in less compression. When weight is applied, sandy soils tend to shift rather than compact, making them preferable for quick drainage projects. For farmers, sandy soils can mean faster germination because they warm up quicker. However, they often lack the ability to retain moisture and nutrients, leading to challenges during dry spells.
Benefits:
- Quick drainage, beneficial in preventing waterlogging.
- Warms up faster than other soils, promoting early plant growth.
Disadvantages:
- Requires more frequent watering to keep crops healthy.
- Nutrient-holding capacity is lower, necessitating regular amendments.
Silty Soils
Now, silty soils fall somewhere in between clay and sandy soils regarding characteristics. They have a finer texture than sand but are coarser than clay. Silty soils are noted for their smooth feel and higher fertility compared to sandy counterparts. They can retain moisture while also allowing good drainage, making them wonderful for agriculture. But they can also be prone to compaction due to their smaller particles, and this can lead to issues such as surface crusting that affects seedling emergence.
Benefits:
- Good nutrient retention, leading to fertile cropping conditions.
- Balanced drainage capabilities.
Disadvantages:
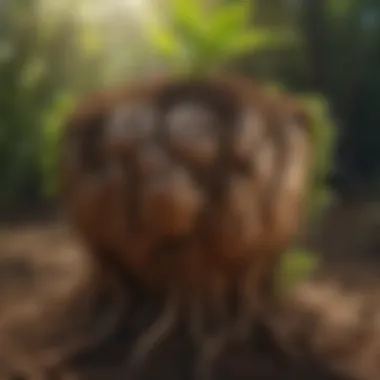
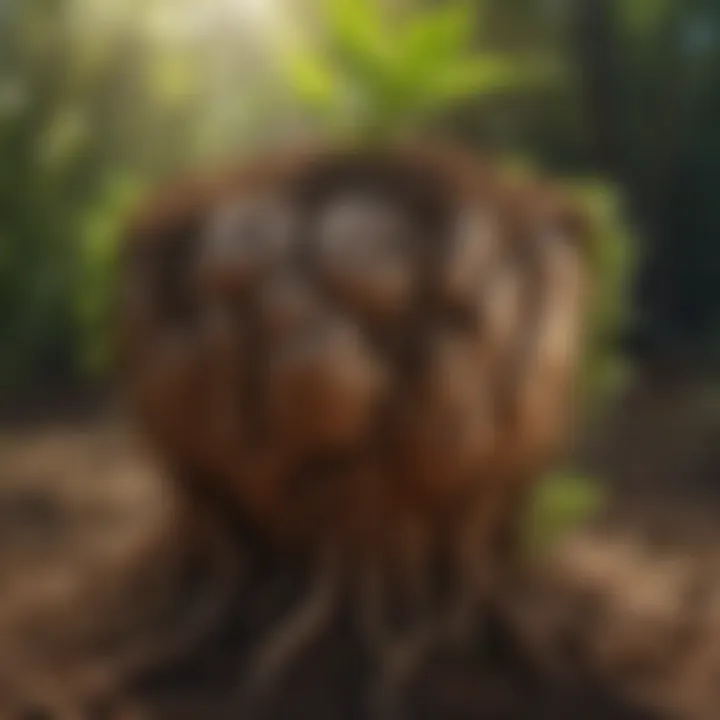
- Can become compacted under heavy loads.
- Susceptible to erosion, especially when left bare.
Role of External Factors
Weight of Structures
The weight of structures is a crucial element in understanding soil compression. When a building or any heavy object is erected, it exerts downward pressure on the soil beneath it. The unique feature of this factor is that the type and distribution of weight can determine how effectively soil compresses. For instance, evenly distributed loads can minimize excessive compression in localized areas.
Benefits:
- Understanding weight distribution helps in designing foundations that minimize soil impact.
Disadvantages:
- Poorly designed structures can lead to uneven compression, potentially causing cracking or tilting.
Water Content
Water content is another significant external factor. Moisture affects how soil particles interact with each other. Wet soils can compress more readily, while dry soils may remain more stable. The key characteristic here is the soil's moisture saturation point. When soil is saturated, it can lose its strength, leading to potential failures in agricultural settings or under infrastructure.
Benefits:
- Properly managed water content can enhance soil's load-bearing capabilities.
Disadvantages:
- Excess moisture can lead to compaction, increasing the risk of flooding in low-lying areas.
Organic Matter
Lastly, organic matter plays a pivotal role in soil structure and its behavior under stress. As organic material decomposes, it binds soil particles together, enhancing nutrients and soil structure, making it less prone to compaction. Thus, a soil rich in organic content can absorb more weight and withstand greater pressure.
Benefits:
- Improves soil aeration and moisture retention, creating better planting conditions.
Disadvantages:
- A lack of organic matter can lead to weak soil structure, affecting resilience against compression.
Impacts of Soil Compression
The phenomenon of soil compression carries implications that touch nearly every aspect of agricultural practices, from crop yields to infrastructure durability. Recognizing the impacts of soil compression is essential, not only for farmers but also for civil engineers and environmentalists. This section dives into how soil compression affects three key areas: plant growth, infrastructure stability, and environmental ecosystems.
Effects on Plant Growth
Root Development
Root development is a cornerstone of a plant's life; think of it as the foundation of a house. Overly compacted soil restricts root expansion, leading to weaker plants. When roots struggle to penetrate compacted layers, it restricts their access to water and nutrients essential for growth.
Key characteristic: Root depth and spread. When soil isn't too dense, roots can go deep into the ground, tapping into moisture pockets during dry spells. This contributes to the resilience of the plant in facing adverse weather conditions. In less favorable conditions, this characteristic equips roots to better withstand droughts or flooding.
One unique feature is the ability of roots to adapt to varying levels of soil texture. For instance, in sandy soils, roots may spread horizontally instead of vying for depth due to compaction challenges. While this strategy might work, it limits the plant's nutrient absorption capabilities, creating a trade-off that farmer must navigate.
Nutrient Availability
Nutrient availability plays a vital role in shaping what crops can grow successfully. Compacted soil can become impenetrable for essential nutrients and water. Nutrients need a pathway to reach roots, but soil compression makes it akin to pushing a boulder uphill.
Key characteristic: Soil porosity. In well-aerated soil, nutrients travel freely to plants' roots. However, excessive compaction reduces air pockets necessary for healthy microbial activity that decomposes organic matter into usable nutrients. This characteristic makes loose soil a popular choice among farmers, as it ensures optimal nutrient uptake by crops.
A unique feature of nutrient availability is its dependency on the microbial ecosystem within the soil. A diversity of microbes is required to enrich nutrients in the soil profile. When compaction occurs, the microbial community may shift, possibly favoring less effective organisms. The result could lead to nutrient deficiencies in plants, which could spiral into lower crop yields.
Consequences for Infrastructure
Building Stability
Building stability hinges significantly on the ground below. Compacted soil can alter the load-bearing capacity of foundations, resulting in issues like settling or even structural failure. Engineers often face the hurdle of ensuring soil can support the weight above it.
Key characteristic: Load distribution. When soil is well-compacted, it distributes weight evenly across a wide area. This helps prevent localized sinking or cracking, which can lead to costly repairs. Hence, a well-understood compaction strategy can be vital for the success of construction projects.
One major advantage of building on well-compacted soils is longevity. Structures that rest on stable soil tend to have extended lifespans, minimizing the need for ongoing maintenance. However, it can also be a double-edged sword; overly compacted soil can lead to poor drainage, resulting in water trapping and further complications.
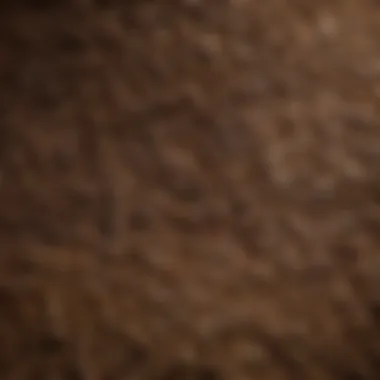
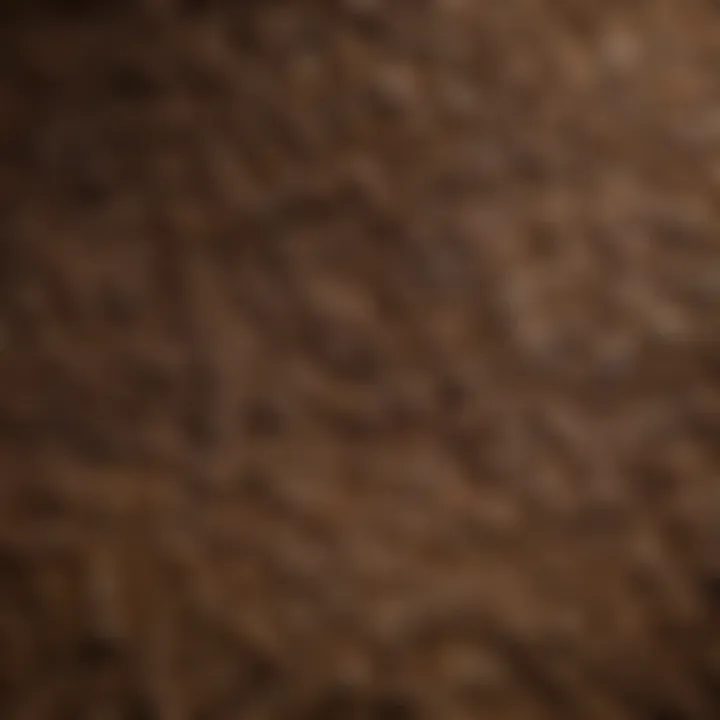
Road Construction
Road construction doesn’t merely involve laying asphalt; it's about creating a firm base. Soil compression directly impacts the quality and durability of roadways. Compacted soil maximizes structural integrity, allowing it to withstand the weight of vehicles and machinery traversing it.
Key characteristic: Stability under load. Well-compacted layers enhance the load-bearing capacity of the road surface significantly. This is crucial, particularly in regions experiencing heavy traffic or extreme weather conditions. Roads that sag or crack are troublesome , leading to increased costs of repairs and maintenance.
One unique aspect of road construction is its reliance on moisture content within the soil matrix. Too much moisture can lead to undesirable effects, like potholes and ruts forming, which can turn roads into obstacle courses. Conversely, if soil is too dry during compaction, it might not settle properly, and in turn, create future hazards.
Environmental Ecosystem Considerations
Soil Erosion
Soil erosion can become a fierce adversary for agricultural practices. When soil is compacted, it becomes less stable, making it more susceptible to being washed or blown away by natural forces. A low-lying area with compacted soil can lead to detrimental cascading effects on neighboring ecosystems.
Key characteristic: Surface runoff. With compacted soil, the water doesn’t seep in as it should but rather flows across the surface, taking valuable topsoil with it. This results in diminished soil quality and can lead to loss of nutrients necessary for plant growth, compounding the challenges for farmers.
A notable disadvantage of erosion is its potential for long-term consequences. Once topsoil is lost, it can take years, if not decades, to rebuild that critical layer. Addressing the erosion with cover crops or implementing no-till agriculture methods can mitigate impacts, but the challenge remains persistent if soil compression is not managed effectively.
Water Runoff
Water runoff is the immediate consequence of compacted soils. When soil is unable to absorb water quickly, it leads to an increase in runoff, straining water systems and damaging surrounding habitats.
Key characteristic: Infiltration rate. Healthy, loosely packed soil allows for greater infiltration rates, promoting groundwater recharge. On the flip side, compacted soils act like a barrier, leading to flooding in some areas while depriving others of needed moisture.
One unique feature of concerning runoff is the increased risk of contamination. Excessive runoff can lead pollutants from roads or fields into water bodies, which creates a strain on aquatic ecosystems. A key consideration is that effective soil management not only enhances agricultural viability but also supports environmental health on a broader scale.
In summary, understanding the various impacts of soil compression is not merely an academic exercise, but a necessity for anyone involved in agriculture, infrastructure, and environmental management. Each component connects back to optimal soil conditions, affecting everything from root growth in crops to the stability of the structures we build.
Assessing Soil Compression
Understanding how to assess soil compression is crucial for anyone involved in agriculture or land management. The methods used to evaluate soil compression can lead to better cultivation practices, improved infrastructure integrity, and overall enhanced productivity in various settings.
When it comes to agriculture, accurate assessment can prevent detrimental impacts on crop yield. Overly compacted soil can restrict root growth and limit nutrient uptake. For infrastructure projects, accurately gauging soil compression ensures that structures such as roads, bridges, and buildings maintain their integrity over time, reducing the risk of costly repairs or even catastrophic failures.
Field Methods
Field methods for assessing soil compression often involve direct observation and in situ testing techniques. These methods provide immediate data about the soil condition. Common techniques include:
- Penetrometers: Used to measure soil resistance, offering a straightforward way to assess compaction levels. Using these devices, one can easily identify compacted layers that hinder plant growth.
- Core Sampling: Involves extracting soil samples to analyze density and porosity. This method can provide insights into how compressive forces have altered the soil structure over time.
- Visual Assessment: Sometimes, a trained eye is all that's needed. Observing indicators such as crop health, soil cracking, or changes in surface texture can give clues about the underlying compaction issues.
Each of these field methods has its advantages and limitations, but they are all effective in providing a snapshot of soil health and conditions.
Laboratory Testing Techniques
Laboratory testing techniques allow for a more comprehensive analysis of soil compression. These methods provide deeper insights compared to field methods, proving invaluable for research and detailed assessments.
Consolidation Tests
Consolidation tests measure how soil compresses over time under various loads. This method is particularly significant because it simulates real-world conditions where soil is subjected to different pressures from above, such as buildings or heavy machinery.
- Key Characteristic: The ability to replicate long-term loading scenarios.
- Why it's Beneficial: By identifying how much soil can compress under stress, researchers and practitioners can make informed decisions regarding site selection and structural design.
- Unique Feature: Consolidation tests usually involve a sample being placed in a specific apparatus, where it is subjected to incremental loads. This offers a clear, measurable outcome.
- Advantages: Provides valuable data on settlement predictions and soil behavior.
- Disadvantages: The need for precise equipment and experienced personnel can make it costly and time-consuming.
Shear Strength Analysis
Shear strength analysis delves into how well soil supports loads without sliding. This aspect is critical in determining how layers of soil will behave under pressure. A solid understanding of shear strength can inform decisions about both agriculture and construction.
- Key Characteristic: It assesses the soil's internal friction and cohesion, factors vital for stability.
- Why it's Beneficial: By determining shear strength, one can evaluate the suitability of soil for various applications, like farming or constructing foundations.
- Unique Feature: Various tests, such as the triaxial test or unconfined compressive strength test, can yield different insights into soil behavior under stress.
- Advantages: Helps in predicting potential failures and ensuring safety in construction projects.
- Disadvantages: Requires specialized equipment and might not be feasible for all types of soils.
Understanding the techniques available for assessing soil compression equips farmers and engineers alike with the tools necessary to enhance productivity and safety in their projects.
Mitigating Soil Compression
Mitigating soil compression is essential for ensuring sustainable agricultural practices and maintaining soil health. To prevent soil compaction from taking a toll on crop yields and land viability, farmers and agricultural professionals must implement various strategies. Understanding the methods available for reducing soil compression can lead to healthier crops, better drainage, and more resilient ecosystems.
Several components contribute to effective mitigation, including choosing best agricultural practices, employing cutting-edge technological innovations, and following regulatory frameworks designed to protect soil health. Each of these elements plays a vital role in bolstering soil structure and fertility, ultimately enhancing productivity in farming operations.
Best Agricultural Practices
Crop Rotation
Crop rotation stands out as a particularly effective agronomic practice leading to reduced soil compression. This method involves alternating the species of crops grown on a piece of land with each planting season. Not only does it disrupt cycles of pests and diseases, but it also positively impacts soil health.
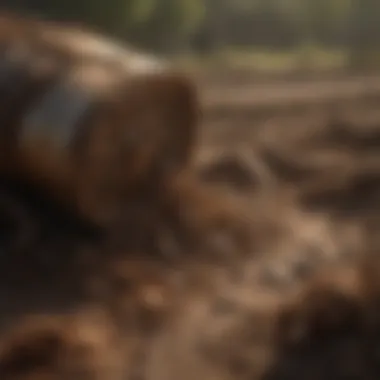
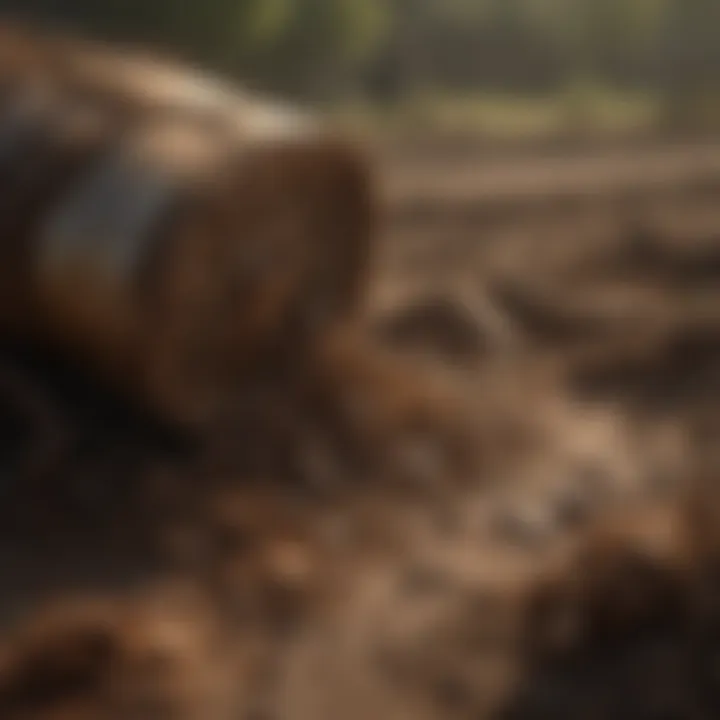
One of the keystones of crop rotation is the introduction of different root structures into the soil. For instance, deep-rooted plants can penetrate compacted layers, alleviating pressure on the soil and helping to improve its structure. This diversity in root systems encourages a variety of microbial life, which is beneficial for soil aeration and nutrient cycling. Because of this, crop rotation is increasingly hailed as a beneficial practice by many in the agriculture sector.
However, a unique characteristic of this approach is its requirement for careful planning. Farmers must take into account factors such as soil type, climate conditions, and market demand when selecting suitable crops for rotation. When done right, crop rotation can lead to long-term soil health, although it may take time for the benefits to materialize.
Cover Crops
Cover crops are another remarkable tool in the farmer's arsenal for mitigating soil compression. These plants, often sown in the off-season, serve multiple purposes, including improving soil structure, increasing organic matter, and enhancing nutrient availability. A critical characteristic of cover crops is their ability to protect the soil from erosion while enhancing water infiltration.
One unique aspect of using cover crops lies in their capacity to fix nitrogen in the soil, contributing to fertility without the need for chemical fertilizers. Leguminous cover crops, for example, enrich the soil, helping subsequent crops thrive.
However, the choice of cover crops must align with the specific needs of a given area. Some crops may require additional management to prevent competition with main crops or pests. Overall, when selected and managed properly, cover crops are an invaluable addition to a sustainable agricultural strategy.
Technological Innovations
Precision Agriculture
Precision agriculture revolutionizes farming practices, offering tailored solutions to mitigate soil compression. Through technologies such as GPS, sensors, and data analytics, farmers can gain insights into soil condition, moisture levels, and crop health. The key characteristic of this technology is that it allows for site-specific management, meaning decisions can be made based on precise measurements rather than blanket assumptions.
For instance, knowing exactly where compacted areas exist allows a farmer to target interventions in those locations only. This not only optimizes resource use but also minimizes the risk of exacerbating issues in adjacent areas. This individualized approach highlights why precision agriculture is increasingly popular among those looking to improve their yields while preserving soil integrity.
Nonetheless, there may be a learning curve when adopting precision agriculture. Initial investments in technology and training can be significant, but the long-term benefits for both soil health and crop production often outweigh these upfront costs.
Compaction Reduction Equipment
Compaction reduction equipment, including specialized tillage tools and subsoilers, is another innovative approach to combating soil compression. These machines are designed to break up compacted soil layers without disturbing the overall soil structure too much. A distinguishing feature of this equipment is its precision; it can target compacted zones directly, leading to greater effectiveness.
Using compaction reduction equipment can enhance aeration, water infiltration, and root penetration, ultimately benefiting plant growth. However, farmers must be cautious about over-tilling or using inappropriate machinery which could lead to further soil degradation. Balancing the use of these tools with careful planning is essential to maximize their effectiveness while protecting soil health.
Investing in appropriate methods to mitigate soil compression can significantly influence agricultural productivity and sustainability standards.
In summary, effectively mitigating soil compression calls for an integration of best agricultural practices with technological innovations. Both crop rotation and cover crops illustrate how natural methods can foster soil health, while precision agriculture and compaction reduction equipment demonstrate how modern technology can support these efforts. This comprehensive understanding empowers farmers to cultivate their land responsibly, creating a healthier and more productive agricultural ecosystem.
Regulatory Framework and Standards
Understanding the regulatory framework surrounding soil compression is crucial for effective management and sustainable agricultural practices. Regulations serve as guidelines to ensure that agricultural activities do not negatively impact soil health and consequently the ecosystem. These frameworks outline requirements that farmers and land managers need to follow to protect soil integrity and promote sustainable practices. They also catalyze innovations in soil management that can lead to better agricultural outcomes.
Local Agricultural Policies
Local agricultural policies play a vital role in defining how soil compression is managed in specific regions. These policies focus on:
- Soil Health: They may promote practices that enhance the soil's structure and minimize compression. For instance, some regions encourage the use of no-till farming techniques that can help preserve soil integrity.
- Support for Farmers: Often, these policies include incentives for farmers who adopt sustainable practices. This could be in the form of subsidies or technical support for using equipment that reduces soil compaction.
- Monitoring and Regulation: Local authorities often conduct soil assessments to find out how different farming practices affect soil health. Regular monitoring helps in making adjustments to policies so that they remain effective in addressing the prevailing challenges.
A good example of a local policy is the promotion of cover crops, which helps in maintaining soil structure, reducing compaction, and improving overall soil health.
International Guidelines
In addition to local regulations, international guidelines provide a broader context for managing soil compression. These global standards aim for:
- Sustainability: Recommendations from organizations like the Food and Agriculture Organization (FAO) emphasize practices that support long-term soil fertility and health on a global scale.
- Research Collaboration: International guidelines encourage collaboration among nations to share knowledge and techniques for mitigating soil compression. These collaborations often lead to enhanced research on the relationship between soil management practices and crop yields.
- Shared Best Practices: Countries may adopt a framework that outlines best practices for sustainable agriculture, which includes guidelines on minimizing soil compaction and encouraging soil recovery practices.
"Sustainable soil management is the foundation of food security and environmental protection. Failing to recognize the importance of soil health can have dire consequences for ecosystems and human livelihoods."
Future Directions in Soil Compression Research
The research regarding soil compression is at a pivotal juncture. With the looming challenges posed by climate change, urban development, and increased agricultural demand, understanding soil compression is crucial to sustainability. Exploring future directions helps pinpoint advancements that can transform agricultural practices and mitigate negative outcomes. This section zeros in on emerging technologies and sustainable practices that hold promise for the field.
Emerging Technologies
As technology marches on, it paves the way for innovative methods to assess and manage soil compression. One main shift is the integration of remote sensing technologies, which gather data on soil health without being intrusive. For instance, satellites equipped with multispectral imagery allow for wide-scale monitoring of soil condition. This can lead to precision agriculture, in which farmers make decisions based on data specific to their land rather than relying on broad generalizations.
Another significant advancement involves soil sensors that continuously monitor moisture levels, temperature, and density. These gadgets can relay real-time information, making it easier to manage irrigation and apply corrections as needed. Such tools not only help in maintaining optimal soil conditions but also contribute to minimizing waste and saving resources.
Furthermore, there is a surge in the development of bioengineering solutions, such as utilizing beneficial microbes that enhance soil structure and health. These biotechnologies can enable soils to better absorb and manage loads, ultimately reducing compression-related issues.
> "Advanced technologies are shifting the paradigm in our approach to soil management, leading us towards more informed, sustainable practices."
Sustainable Practices for the Future
In the context of soil compression, sustainable practices play a vital role in addressing some of the related challenges. One highly advocated method is agroecology, which emphasizes the symbiotic relationship between agriculture and natural ecosystems. Practices like intercropping and polycultures not only improve soil stability but also aid in fostering biodiversity.
Another commendable approach involves using less intensive machinery or even advancing towards manual cultivation where possible. This can significantly reduce soil compaction, allowing for better root penetration and water retention. Additionally, reducing the weight of machinery during the farming process can ease the pressure on soil, maintaining its integrity.
Cover crops also serve as a protective measure against compression. These crops, planted during the off-season, not only cover the ground but can also improve soil structure through root systems. They contribute to retaining moisture and nutrients, helping minimize soil erosion and compression effects.
The adoption of organic amendments—like compost or biochar—also shows promise. These materials enhance soil aeration and structure, thus providing better load-bearing capacity and improving organic matter content.
By focusing on such sustainable practices, future research can outline a roadmap of actionable steps that not only address current issues relating to soil compression but set a foundation for regenerative agricultural practices that benefit both farmers and the ecosystems they operate within.